SCK/CEN dates from 1952, and in the course of its history fielded four nuclear research reactors, of which three remain operational.
Soon, a new one will be added, but a completely different one: MYRRHA.
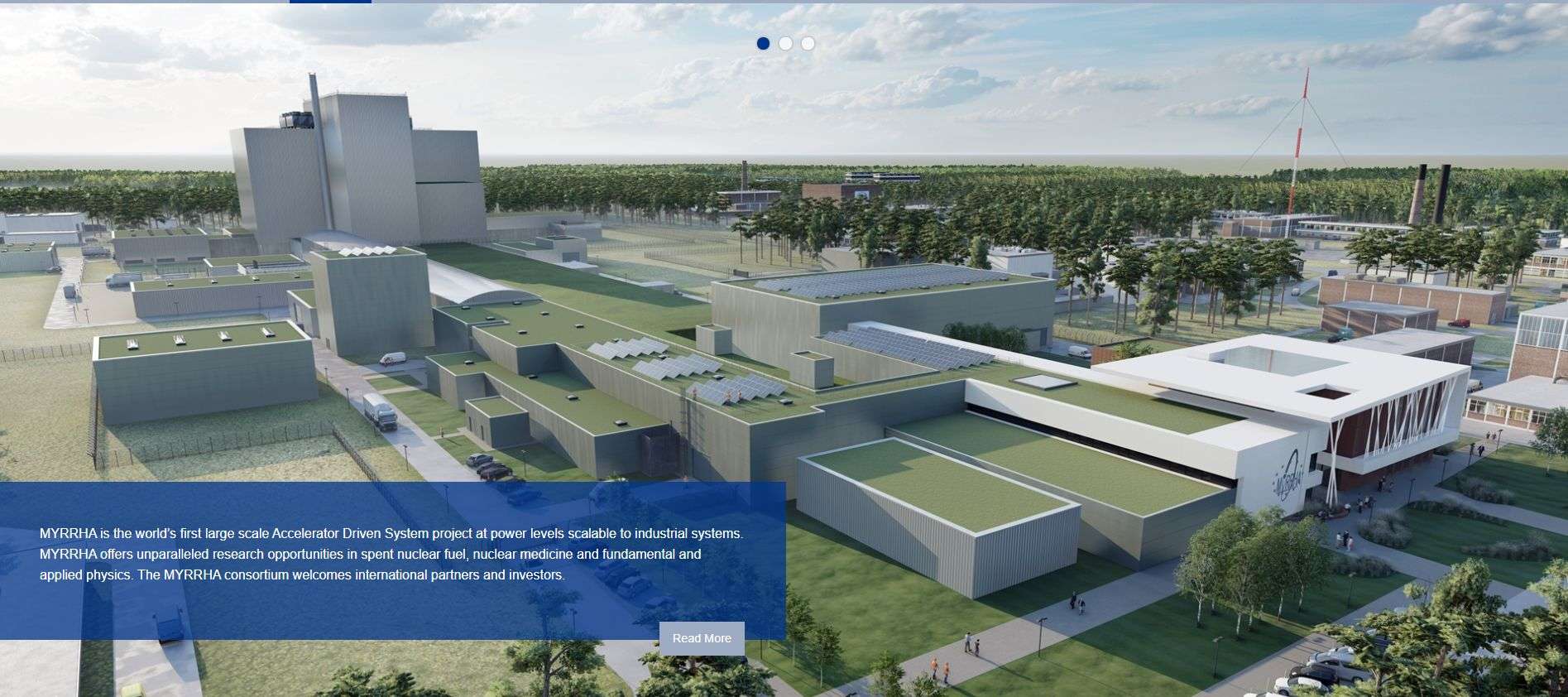
MYRRHA, which got the green light from the Belgian government on SEP 7, 2018 with a budget of € 1.6 bn, stands for Multi-purpose hYbrid Research Reactor for High-tech Applications. It will be the world’s first large scale Accelerator Driven System (ADS), consisting of a subcritical nuclear reactor driven by a high power linear accelerator (linac). Because of the subcritical concentration of fission material, the nuclear reaction is sustained by the particle accelerator only (this means that turning off the proton beam results in an immediate and safe halt of the nuclear reactions).
Before going into details re the MYRRHA reactor, first some quick recap on the kind of nuclear fission in thermal neutron reactors, which constitute the bulk of our nuclear powerplants. Namely fission of supercritical mass, i.e. fission material in which a nuclear reaction, once started, is self-sustaining. As the pic shows, a neutron penetrates a Uranium U-235 isotope, which absorbs it, thus leading to an isotope with a larger 'mass', U-236 (I assume you know that we are still talking about a uranium atom, as the number of protons remains the same):

U-236 is unstable and quickly falls apart in two lighter cores (often Krypton and Barium, but other combinations happen as well) PLUS two or three neutrons, which go on to bump into other U-235 atoms, thereby also causing them to split. The multiplication factor k, is defined as the number of neutrons in one generation divided by the number of neutrons in the preceding generation, and it is clear that if k is greater than 1, the chain reaction is indeed self-sustaining (supercritical): the neutron population will grow exponentially.
If k is less than 1, the chain reaction is subcritical, and the neutron population will exponentially decay - the nuclear chain reaction will stop in an of itself.
Every time a heavy uranium atom falls apart, an energy amount of roughly 200MeV is released. In practice this boils down to a kilogram of uranium-235 (U-235) yielding approximately three million times more energy than a kilogram of coal burned conventionally (7.2 × 1013 joules per kilogram of uranium-235 versus 2.4 × 107 joules per kilogram of coal).
Now, to initiate nuclear fission in thermal neutron reactors, it usually suffices to place a so-called startup neutron source, e.g. Californium-252, in the reactor core loaded with fresh nuclear fuel, e.g. UO2 or MOX (for Mixed Oxides, meaning a mixture of UO2 and PuO2). The neutron flux in UO2 or MOX is in and of itself insufficient to trigger a nuclear reaction, but adding certain quantities of this startup neutron source will do the trick, and a smooth and reliable initiation of fission will take place.
It is completely different in the type of reactor that MYRRHA is about. First of all, in such a reactor, an "Accelerator Driven System', the nuclear fuel is subcritical, meaning that it cannot sustain a chain reaction itself. But secondly, the 'bombarding' neutrons do not generate from a 'startup neutron source' but originate instead through a mechanism called 'spallation'. The diagram below shows how a powerful proton beam is 'shot' in a 'spallation target' encapsulated by subcritical fuel:

The following pic offers some more specifics for SCK's ADS:
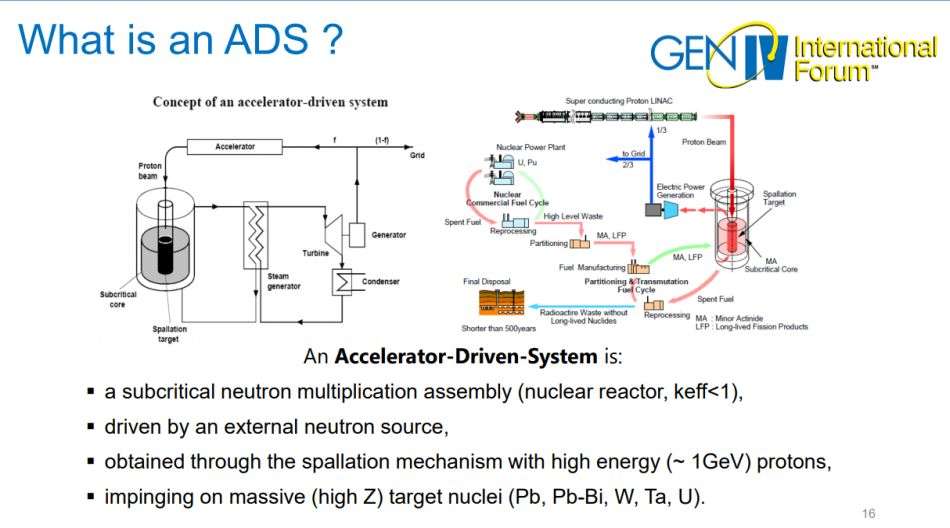
Now, Before we go any further with this, it's useful to illustrate the spallation phenomenon using an appropriate analogy, and what better analogy than spall in AFV's?
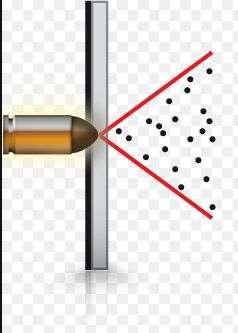
Even if a round does not penetrate an AFV's armour, it may cause tiny pieces, flakes etc to break free from the armour plate's other side - i.e., the AFV's interior - to break off the main body and go flying through the inside of the vehicle, thereby causing potentially serious injury to the crew. Particulary in WW1, with the techniques of producing decent armour plates still in their infancy, and no one yet thinking of installing spall liners, this was a serious problem, leading to the adoption of so-called splatter masks:
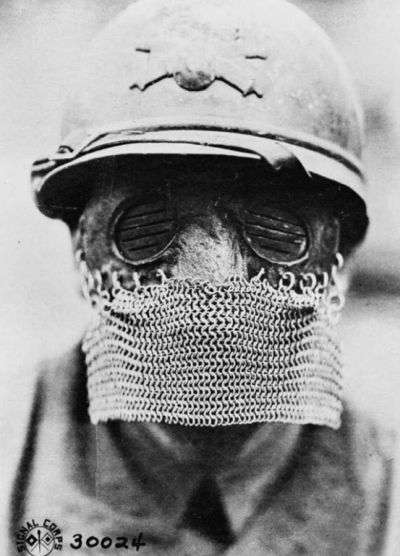
Now, imagine that instead of a shell striking an armour plate, a proton at nearly the speed of light slams into an atom:
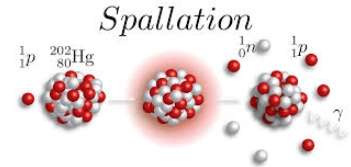
A particle beam consisting of protons at around 1 GeV is shot into a so-callad 'spallation target' consisting of mercury, tantalum, lead or another heavy metal. A 'cloud' of subatomic particles scatters, much like metal flakes and fragments are ejected upon impact of the shell in the example above. As protons too are dispersed, the element effectively becomes another one of lower atom number.
Although it's actually a bit more complicated than that, because...

... spallation can best be imagined as a two-stage process. In the first stage, the high-enery proton reacts with nucleons-neutrons and protons-inside the nucleus. The reactions that follow cause an 'intranuclear cascade' of high-energy (greater than 20 MeV) protons, neutrons, and pions within the nucleus. During this intranuclear cascade, some of these energetic hadrons escape as secondary particles.
Others transfer their kinetic energy in the nucleus leaving it in an excited state. In the second stage (nuclear de-excitation), so-called evaporation takes place when the excited nucleus relaxes by emitting low-energy (less than 20 MeV) neutrons, protons, alpha particles, etc., with most of the particles being neutrons. The low-energy neutrons produced during evaporation are important in a spallation source because they can be moderated (reduced) to even lower energies for use as research probes. After evaporation, the nucleus that remains may be radioactive and may emit gamma rays.
Secondary high-energy particles produced during the intranuclear cascade move more or less in the same direction as that of the impacting proton and can collide with other nuclei in the target. The reactions that follow are a series of secondary spallation reactions that generate more secondary particles and low-energy neutrons.
To sum it up, spallation is a nuclear reaction in which a 'spallation target' (ST) is bombarded by very high-energy (> 50MeV) particles. The incident particles, such as protons, disintegrate the nuclei through inelastic nuclear reactions. The result is the emission of protons, α-particles, and mostly neutrons.
So how do neutrons originating from fission differ from those resulting from spallation? One difference is in the nuclear debris remaining after the reactions. But the most important difference between spallation and fission neutrons is the number released. The overall number of neutrons resulting from one fission event (about 2.5) is considerably less than that resulting from a spallation event, for about 13 neutrons for each incident proton may be released.
And this is where the concept of the Accelerator Driven System, or better, Accelerator Driven Subcriticial Reactor, in a recent past referred to as 'Energy Amplifier', comes into play. The idea is the brainchild of renowned nuclear scientist Carlo Rubbia, who proposed a nuclear reactor based on a proton cyclotron accelerator with a beam energy of 800 MeV to 1 GeV, and a target with thorium as fuel and lead as a coolant. Its advantages were and are manifold:
1.) "Subcritical" means that the reaction could not run away — if anything went wrong, the reaction would stop and the reactor would cool down.
2.) Thorium is an abundant element — at least much more than uranium — reducing strategic and political supply issues and eliminating costly and energy-intensive isotope separation. There is sufficient thorium to generate energy for at least several thousand years.
3.) This kind of reactor would produce very little plutonium, thus more "proliferation-resistant" than conventional nuclear power.
4.) The reactor offers the perspective of "consuming" plutonium, reducing the world stockpile of the very-long-lived element.
5.) Less long-lived radioactive waste is produced — the waste material would decay after 500 years to the radioactive level of coal ash.
6.) Building ADS reactors requires primarily an engineering effort, since the science behind the concept is established and more (fundamental) reseach is not needed.
7.) Power generation might be economical compared to current nuclear reactor designs if the total fuel cycle and decommissioning costs are considered.
8.) The design can work on a relatively small scale, and has the potential to load-follow by modulating the proton beam, making it more suitable for countries without a well-developed power grid system.
9.) Inherent safety and safe fuel transport can make the technology more suitable for developing countries as well as in densely populated areas.
And here we come back to SCK's MYRRHA project, which will consist of four major components, to be constructed in three phases:
1.) Phase 1: design and construction of the first linac section (up to 100 MeV). Testing this section will show whether the linac is up to the operational reliability required to drive the reactor with a 600 MeV proton beam. Additionally, Phase 1 will also encompass the Proton Target Facility for the production of medical radioisotopes and for fundamental and applied research in physics as well as for material research. A third component is the Fusion Target Station, where materials for fusion reactors will be tested. This phase is scheduled for completion in 2026.
2.) Phase 2: extension of the 100 MeV linac to 600 MeV. This extension is required to drive the reactor. When completed, the linac will be approximately 400 m long. Phase 2 is scheduled for completion in 2033.
3.) Phase 3: construction of the reactor. The double wall, unpressurised pool-type vessel will accommodate all primary systems. The reactor is scheduled to be commissioned in 2036.
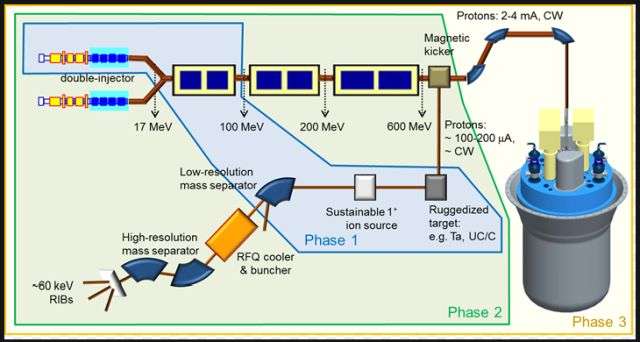
A.) THE LINEAR ACCELERATOR (LINAC).
A 400 m long linear accelerator (linac) will provide protons at an energy of 600 MeV to the reactor. In contrast to Rubbia's original proposal, a linear accelerator was selected due to its enhanced beam stability when compared with cyclotrons. Reliability as an essential performance goal for MYRRHA is reflected in the design of the linac, which boasts twin redundant 17 MeV injectors. The remaining part of the accelerator up to 600 MeV based on super-conducting radio-frequency modules is a common choice in view of optimised performance with high beam currents.
At the end of the linac, the 4 mA proton beam is injected into the reactor, generating a flux of fast neutrons through spallation. Simultaneously, protons are also fed to the multi-purpose Proton Target Facility and to the Fusion Target Station. Currently, the injector is being assembled and tested at the Cyclotron Resource Centre (CRC) at UCLouvain in Louvain-la-Neuve in Wallonia.
By and large, construction of the injectors and the first linac stage (up to 100MeV) constitues the first phase in the project, to be concluded by 2026. However, SCK being first and foremost a, nuclear research facility, Phase 1 will also incorporate building a Proton Target Facility for the production of medical radioisotopes and for fundamental and applied research in physics, as well as for material research.
B.) THE LEAD-BISMUTH EUTECTIC (LBE) COOLED REACTOR.
The MYRRHA reactor is so-called pool type, cooled by 7,800 tons of lead-bismuth eutectic (LBE). LBE is a eutectic alloy, meaning that it melts or solidifies at temperature lower than the melting point of any of the constituents (LBE consists of lead (44.5%) and bismuth (55.5%) and has a melting point of 123.5 °C (whereas pure lead melts at 327 °C and pure bismuth at 271 °C).
All primary systems are within the double walled 16 m high and 10 m wide reactorvessel:

It's a rather crowded pic, so to dinstinguish the LBE path as it is pumped down, picks up heat from the core, is pushed upwards again where it transfers its heat to water in the blue colored heat exchangers, this pic may help...
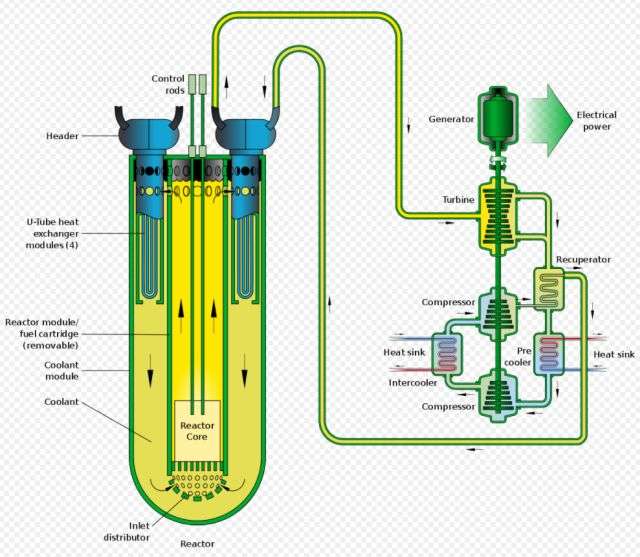
...while this schematic offers some more insight re the disposition of the actual core:

This is the proposed layout of the core (via a pdf by Prof. Dr. Hamid Aït Abderrahim, Director of MYRRHA and Deputy Director-General of SCK CEN).
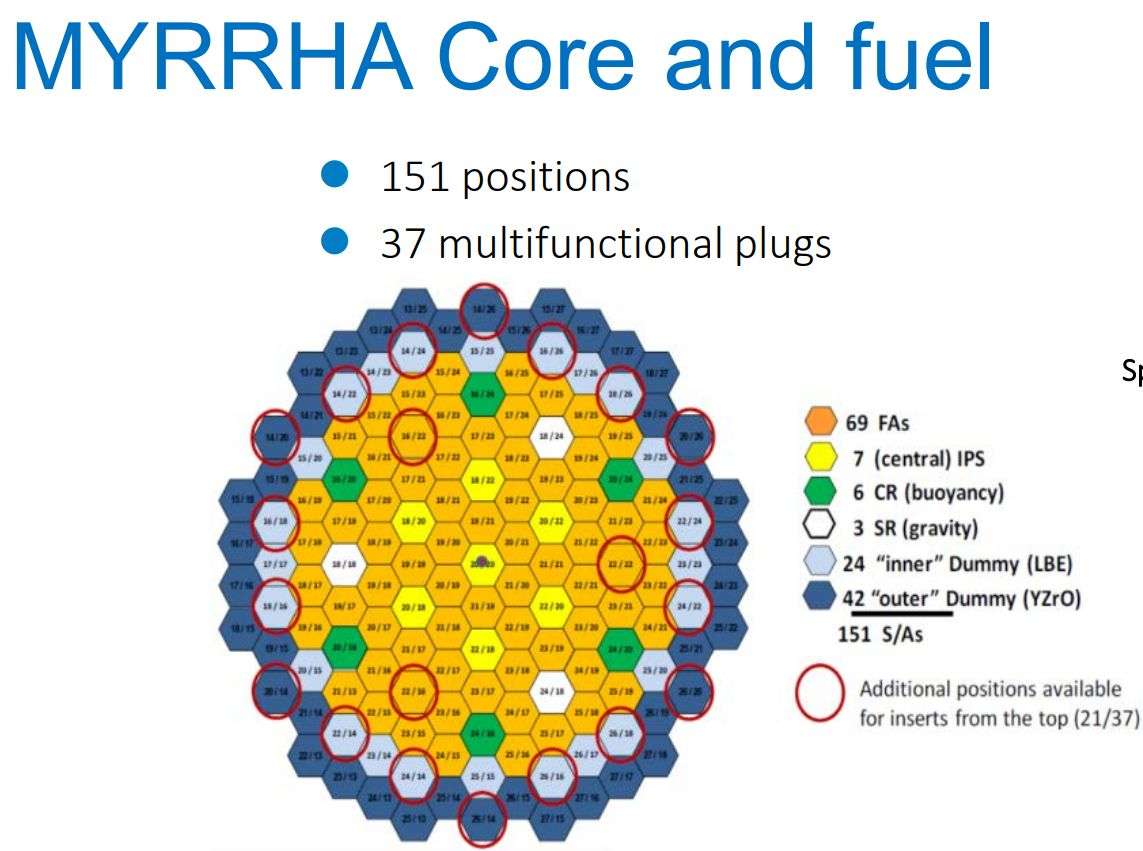
Some more detail re the layout is provided by this schematic, per Didier J. De Bruyn:
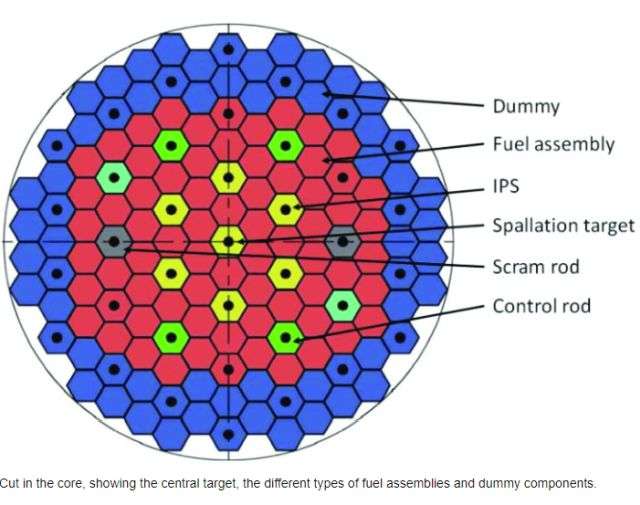
At the design's present state, the reactor core consists of mixed oxide (MOX) fuel pins, typical for fast reactors. The In-Pile test Sections (IPS), which will be located in dedicated fuel assembly (FA) positions, are larger in diameter giving more flexibility for experiments. Thirty seven positions can be occupied by IPSs or by the spallation target (the central one of the core in sub-critical configuration) or by control and shutdown rods (in the core critical configuration). This gives a large flexibility in the choice of the more suitable position (neutron flux) for each experiment.
A final 3D-schematic of the core:

To cap it off, a list of the advantages offered by the use of LBE in the MYRRHA reactor:
* Wide operating temperature range: LBE has a relatively low melting point (125 ºC) when compared with its individual components (lead 327 ºC, bismuth 271 ºC), while it boils only above 1670 ºC.
* Excellent heat conductivity: LBE enables the reactor’s primary system to operate under normal pressure.
* Radiation shielding: LBE is an excellent radiation shield that blocks gamma radiation.
* Virtually transparent to neutrons: LBE is an ideal medium as spallation source inside the reactor where the linac’s proton beam is converted into neutrons required to sustain the fission reaction.
* Fuel mix: LBE allows a wide variety of fuels to be used besides uranium 235 and 238, including mixed oxide (MOX) fuels. In particular, simulations indicate that linac driven, LBE cooled reactors may contain up to 30% of long-lived minor actinides, such as neptunium, americium and curium.
* LBE based reactors use far less nuclear fuel and produce far less nuclear waste.
By default, the MYRRHA reactor has a sub-critical design: it doesn’t contain enough fissile material to maintain the chain reaction (although it is capable to operate in a critical mode!). An additional safety factor is the reactor's passive cooling capacity: in case of electric failure, or when the linac is switched off and the reactor comes to an immediate stop, the cooling of the reactor is further guaranteed by the natural circulation of the LBE.
MYRRHA is projected to have a maximum output of 100 MWth (MW for thermal energy).
C.) THE PROTON TARGET FACILITY.
The Proton Target Facility (PTF) is a multi-purpose research infrastructure that will use up to 0.5 mA of the 100 MeV proton beam. It will ensure the production of radioisotopes and research into several fields. Radioisotopes, short for an element's radioactive isotopes, are essentially atoms that contain an unstable combination of neutrons and protons, or excess energy in their nucleus. They are used in the medical sector as radiopharmaceuticals for diagnostic or therapeutic ends, in industrial radiography, which uses a gamma source to conduct stress testing or check the integrity of welds (e.g. to test aeroplane jet engine turbines for structural integrity), and in scientific research, e.g. tracing the flow of contaminants in bilogical systems.
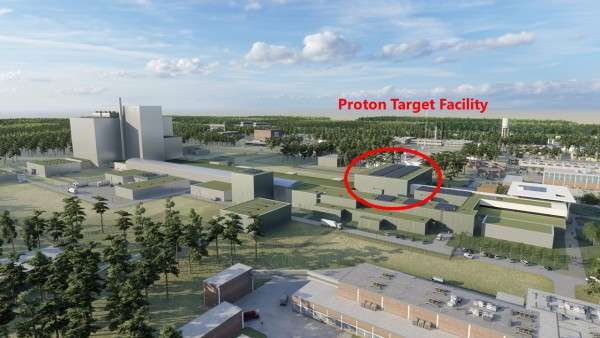
The PTF's "heart" is the Isotope Separation On-Line (ISOL) system, where a wide range of radioisotopes will be produced by nuclear reactions of the proton beam with the primary target material (a.o. ceramic materials containing uranium, thorium or tantalum). The ISOL-produced radioisotopes are extracred from the target in a delicate and technologically challenging task, involving lasers, electrostatic devices (quadrupoles and electrodes) and precisely tuned magnetic dipoles. Finally, one specific radioisotope with high purity will be transported to the laboratory for further manipulation. Radioisotope manipulation can range from a pure collection onto a foil (for medical isotope harvesting for instance) to trapping them in electromagnetic “bottles” and observing the radioactive decay in a very detailed manner.
The full PTF laboratory surrounding the primary target container will consist of:
* a facility for handling and temporary storage of the used primary targets, * a mass separation room, * a radiochemistry laboratory, * a laboratory where various experimental setups for fundamental research can be installed.
The PTF will be available for national and international research groups.
D.) THE FUSION TARGET STATION.
Even though MYRRHA is a research reactor designed to test the feasibility of targeted proton beams to yield neutrons instead of relying on self-sustaining reactions, there's a part of MYRRHA dedicated to research on fusion instead of fission! The Fusion Target Station (FTS) is, indeed, a nuclear fusion oriented research infrastructure designed to handle a proton beam of 4 mA and 100 MeV to irradiate fusion materials.

According to the MYRRHA website, FTS consists of a flowing water containment separated from the vacuum by means of a thin metal window. By adjusting the position in the water tank, the proton beam energy can be fine-tuned to match irradiation conditions necessary to investigate fusion materials. The design will also enable the introduction of a water cooled spallation source that can generate a hybrid proton-neutron irradiation field. In normal people parlance: so the tiny proton beam that is tapped off the main beam will be used for proton irradiation, but a spallation source is also possible, and its resulting neutrons will provide the neutron irradiation part, hence the term 'hybrid proton-neutron irradiation field'.
MYRRHA: "Upon operational deployment, the FTS will be applied to investigate accumulated irradiation damage and/or to perform in-situ testing of materials exposed simultaneously to mechanical load and irradiation beam. Irradiated samples will be transferred to hot cell facilities at SCK CEN or elsewhere, where they can be thoroughly examined with state-of-art equipment. FTS exploitation focus will therefore be on screening perspective/innovative materials and assessment of irradiation-creep softening effects as well as diagnostic equipment validation."
For those interested, Neutron Irradiation of structural materials leads to a variety of atomic-size defects, which generally increase the strength (but can severely reduce ductility). A popular term is Defect-induced strengthening (or radiation hardening). More info here.
Simultaneously, Proton Irradiation has become a very valubale radiation damage tool. Proton Irradiation experiments can capture the effects of neutron irradiation on microstructure, microchemistry, and hardening. Further reading here.
The FTS facility will consist of the following elements:
* an irradiation module * an instrumentation module * a hot cell for transportation and sample reloading * a dedicated testing laboratory in hot cells of Nuclear Material Science Institute
FTS, a research facility within a research facility so to say, will welcome research projects at both the national and international level, and will be tightly linked with European fusion research activities (EFDA/EUROfusion consortium).
This post would not be complete without shedding some light on the crucial role of nuclear physicist Prof. Dr. Hamid Aït Abderrahim, an Algerian native nationalised Belgian, who is so to say MYRRHA's Godfather:
An immensely interesting pdf detailing a webinar by the Professor can be found here.
We wish Prof. Dr. Abderrahim and his team all the best for a successful conclusion of this groundbreaking project!

MFBB.